In all her years conducting research, Narine Sarvazyan, Ph.D., professor of pharmacology and physiology at the George Washington University School of Medicine and Health Sciences (SMHS), has had several ideas, but one — a cardiac imaging catheter — is the closest she’s come to traversing the information chasm between bench and bedside.
“There are still many, many hoops to jump through, but we’re at least approaching [a clinical device],” she says. “It doesn’t really happen very often in basic science research. Usually we think, ‘Well, this might help sometime in the future because we are providing basic knowledge of how things work.’ In this case, I am cautiously optimistic, let’s put it that way.”
Seeing the Invisible
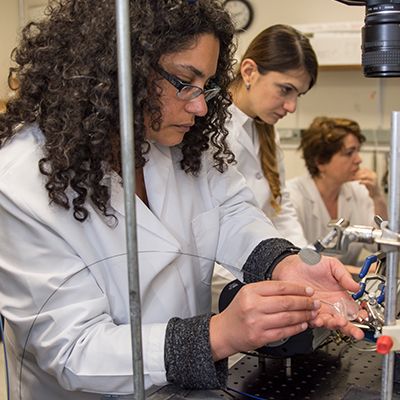
Sarvazyan’s journey began with Marco Mercader, M.D., director of electrophysiology research and associate professor of medicine at SMHS, and Matthew Kay, P.E., D.Sc., an associate professor of biomedical engineering at the GW School of Engineering and Applied Science (SEAS), when the three began experimenting with NADH (a reduced form of nicotinamide adenine dinucleotide) fluorescence imaging on the surface of ischemic animal hearts. “If you illuminate tissue with ultraviolet light, there’s a fluorescence that comes out, and it can be picked up and detected in the laboratory,” explains Mercader. “So Narine and I started experimenting with what would happen if you did ablation of the cardiac tissue to the fluorescence provided by NADH.”
Cardiac ablation, a procedure that burns or freezes heart tissue in an effort to correct arrhythmia, such as atrial fibrillation, comes with its own special set of complexities. Right now, Sarvazyan says, surgeons perform ablation without being able to see what happens to the tissue, which accounts for the current success rate of the procedure: Typically, 60 percent of patients see their arrthymia corrected, and 40 percent return to the hospital for an additional procedure. Out of that number, 10 to 15 percent return one more time.
“There are different means to ablate abnormal sources of electrical activity, but today a surgeon does not really have the means to say, ‘OK, I destroyed the tissue,’ because this procedure is essentially done in a blind fashion,” Sarvazyan says. “There are no direct imaging tools to visualize the ablation process.”
That’s where Sarvayzan’s team comes into play. The team’s goal was to develop a new generation of cardiac imaging catheters that could detect changes in tissue autofluorescence caused by ablation. The current version of their catheter, designed to thread into a patient’s vein and up to the heart, has 17,000 imaging fibers, each capable of picking up a fluorescent signal from the tissue and relaying it to a camera. She compares it to a butterfly eye, capable of using multiple receptors to receive light signals.
In order to see ablation lesions inside the heart, however, it’s not enough to have a small and flexible imaging fiber bundle, Sarvazyan says. Surgeons have to be able to detect damaged tissue beneath thick layers of collagen covering the inner surface of atrial tissue. “The contrast is very, very small,” she says. “So, to be able to reveal damaged tissue, we use technology called ‘hyperspectral imaging.’ ”
Hyperspectral imaging, which has long been used in agriculture and astronomy, is rapidly gaining popularity for clinical applications. With the help of a computer, Sarvazyan explains, it can distinguish individual pixels based on the small spectral differences between them, creating a color map showing ablated tissue and viable gaps still needing ablation.
Sarvazyan and her team also discovered that the intensity of the assigned color was proportional to the depth of the affected tissue. “Our data suggest that when one plots the intensity of the lesion component revealed by hyperspectral imaging, it predicts how deep the lesion is,” she says. “That’s what is known as the holy grail of atrial fibrillation treatment.”
In addition to the imaging fibers, the catheter includes an illumination fiber. “We need to minimize all parts of the catheter for it to fit inside a vein,” Sarvazyan explains. “So from a technical perspective, our main challenge is to get enough light to the tissue to illuminate it, as well as to get enough light back so we can actually spread it into individual wavelengths to do hyperspectral image analysis.” The team’s solution for the current catheter was to envelop the illumination fiber with a pear-shaped saline balloon that acts as a kind of lens, moving blood away from the fibers while lighting the way forward.
Seeking Partnership
While progress ticked along at the bench, Sarvazyan and Mercader, wanting to move their catheter to the translation phase, needed to find financial support. The first phase of the project, Mercader says, was funded by GW and SMHS clinical partner GW Medical Faculty Associates. Then, he adds, came the question: “How are we going to get other people interested in this?”
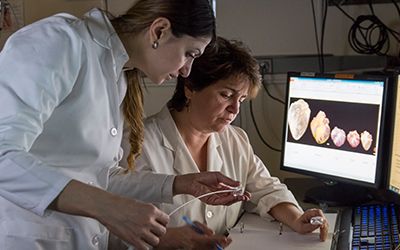
They started by drawing attention to their work. In 2011, the team submitted their idea to the GW Open House and Innovation Competition. They then filed a patent, with Mercader leading the way to find an investor, which he did: venture capital firm Allied Minds. Together, Allied Minds and GW formed a joint company, LuxCath, LLC, which then sponsored a small business, Nocturnal Product Development, LLC. The latter company, essential to the catheter’s development, is an engineering firm specializing in biomedical device design and manufacturing. More recently, the team welcomed Murray Loew, Ph.D., professor of biomedical engineering at SEAS, to help with additional image analysis and processing of algorithms.
Next, with Nocturnal Product Development as their small business partner, Sarvazyan’s team applied for and received a $200,000 National Institutes of Health (NIH) Phase I Small Business Technology Transfer (STTR) Program award in 2014. “Phase I allows one to have the initial funds to prove that the main concept works,” Sarvazyan explains.
The pace of progress slowed when it came to the larger STTR Phase II award. The NIH, Sarvazyan says, is keen on translating whatever researchers find in the lab to the real world, and Phase II of the grant includes elements critical to that goal, such as a marketing plan. “That was certainly uncharted territory for me,” she says, “but we got it done with help from a marketing firm and our business partners.” In April 2015, she submitted the paperwork for the grant, and by July, the team had received a high score on the proposal, successfully checking off each of the criteria.
The NIH, she says, typically awards a Phase II STTR grant two or three times a year. Given her past experience in the grant process and the top marks for her project, Sarvazyan thought the award was a given. At first, “obviously, everyone was ecstatic,” she recalls. “When you get a score like this, you think that you are definitely going to receive the money. But in the fall of 2015, the NIH changed its rules by adding yet another layer of selection for this specific type of grant, which gave us a lot of anxiety.” Sarvazyan was now caught in limbo. “The hardest part was that once you get this score, everyone you call — your chair, your students — they all assume you get the money,” she says. “But then for a year, we were still awaiting the NIH final decision, while trying to keep our team and spirits together. ”
Finally, in August 2016, Sarvazyan received the news. “Luckily for us, the decision was in our favor.”
The two-year grant, totaling $2.27 million, is split between GW and Nocturnal Product Development, with Sarvazyan as the primary investigator. Since the award, the project has moved one step closer to the bedside: On Jan. 31, 2017, the team conducted a large animal study using the first iteration of the hyperspectral imaging catheter. Mercader also traveled to Europe to test an earlier, simpler version of the catheter to get feedback on its functionality.
“We’re so very proud that the catheter made it into an actual catheter; it comes in a box, has a name and a serial number, and it was actually [used] for the first time this year,” Mercader says. The team went to Prague to do first-round testing with both U.S. and local electrophysiologists.
For now, the team is implementing the many tasks outlined in Phase II and preparing for Phase III, which requires a matching investment from a venture capital firm or angel investor to qualify for NIH support. In the meantime, however, Sarvazyan and her team can already lay claim to a major milestone. They are the first at SMHS to receive an STTR Phase II award.
“We believe and hope that this powerful new technology will finally help cardiac surgeons and electrophysiologists to see what was once invisible,” she says.